The Power Of Stars
Nuclear power has a bad reputation with many people. It is partly justified, with disasters like Chernobyl or Fukushima having stained its reputation.
Other people have the opposite opinion, considering that anything short of splitting atoms is a primitive and inefficient way to produce energy. They also point to the low carbon emissions and highly stable baseload power that nuclear energy can provide.
It is likely that nuclear energy will be part of our future energy mix, especially as the 4th generation of nuclear reactors starts to come online, which will be cleaner, safer, and more efficient.
However, all of these reactors rely on the concept of nuclear fission. They take very heavy atoms like uranium, thorium, or plutonium, and collect energy when they break into lighter elements.
Another form of nuclear energy is nuclear fusion. It relies on taking very light elements and making them fuse into heavier ones.
Nuclear fusion is literally what powers the universe, with every star a gigantic nuclear fusion reactor. Every second, the sun consumes 600 million tons of hydrogen. For reference, this means the sun consumes an amount of hydrogen as large as the entire mass of the Earth every 70,000 years.
Funnily enough, it means that solar energy (as well as wind, biomass, and even ultimately fossil fuels) is really just nuclear fusion power (from the sun), except with extra steps.
So, if we could replicate just a small amount of this on Earth, we could access a virtually unlimited energy supply. Contrary to uranium or thorium, which are relatively rare, hydrogen is 74% of all the matter available in the universe.
Fission Vs. Fusion
When we burn molecules like natural gas or oil, we release the energy contained in the chemical bonds of the molecule. This is a rather high level of energy but nowhere near the same range as the energy contained in atoms themselves.
This is why 1 kg of uranium contains the same amount of energy as 2.7 million kg of coal. Hydrogen, when undergoing fusion, is even more powerful.
When discussing nuclear energy, it can be confusing to understand why you can make energy from both fusion and fission.
The reason is that the energy contained in an atom”s nucleus varies depending on the weight of the element. Heavy elements’ nuclei contain more energy than medium-weight elements, so when they split, they release some of that energy as heat and radiation. This heat is what we collect to produce power with nuclear power plants.
But very light elements are even more energetic. So when we merge them into medium-weight elements, they release even more energy.
Source: Nature
As a result, nuclear fusion can produce 3-10x more energy than splitting atoms.
Combined with the extreme abundance of the lightest possible element, hydrogen, this theoretically makes nuclear fusion a source of unlimited power, limited only by the total quantity of matter in the whole universe.
Even in the solar system, the gas giants and the comet clouds contain so much hydrogen that it dwarfs the entire mass of Earth.
Realistically, even a human civilization using 1,000 our current energy consumption would never run out of fuel.
Even better, the resulting product of hydrogen fusion, helium, is a non-toxic, light, and chemically non-reactive gas. So, no nasty nuclear waste to deal with when the process is over.
Fusion Is Hard
Why have we not powered human civilization with nuclear fusion yet?
Well, the thing is that nuclear fusion is hard to achieve. Hydrogen atoms’ nuclei have a positive electric charge and naturally repeal each other. So it can be very hard to get them close enough to each other for fusion, like 2 ultra-strong magnets repealing each other.
In nature, only the crushing gravity of an entire star is enough to push close enough hydrogen atoms to trigger fusion. Even something as big as Jupiter is still “too small” to achieve it.
So, making hydrogen atoms come close together on Earth is very, very hard.
It has, however, been done and was first achieved by fusion machine in the 1950s. These machines demonstrated the feasibility of creating fusion but failed to return enough energy compared to the energy used to trigger the fusion.
(Technically, large-scale nuclear fusion was achieved as early as 1952 with the first thermonuclear bomb, but this is hardly a usable technique for creating a safe power supply).
Another issue with fusion is that nuclear fusion plasma is extremely hot, usually above 100 million Celsius degrees. So it needs to be perfectly contained or it will melt the reactor.
Because of all these problems to solve, nuclear fusion has been a slow-moving field, with the sarcastic comment that “Fusion is always 30 years in the future”.
Replacing Gravity
This issue of creating enough energy back from fusion, compared to the one used to trigger the nuclear fusion reaction, is a recurring one in the field. As fusion is so hard to achieve, compressing even just a few hydrogen atoms is extremely energy-intensive.
Several methods have been proposed so far.
Each has been demonstrated to “work”, meaning that they cause hydrogen or other light elements to fuse into heavier elements and release energy.
Tokamaks
Fusion reactors create a donut-shaped space with magnetic fields, where the nuclear fusion plasma can be contained.
This is currently one of the designs considered to have the highest chance of being optimized into a commercial fusion reactor. The first tokamak was built in 1958 and is the basic concept for ITER (International Thermonuclear Experimental Reactor), the largest research effort to develop commercial fusion, with almost all technologically advanced nations participating in the project.

Source: DOE
However, ITER has been a troubled project with considerable delays. Recently, it was announced that energy-producing reactions might not happen before 2039.
Other Magnets Fusion Reactors
Besides tokamaks, other designs use magnets to compress and confine the plasma. This includes stellarators, spheromaks, and compact tori.
In a stellareactor, the donut shape is irregular/twisted. In theory, it can allow for a longer duration of fusion reactions and a more stable plasma. In practice, it is very hard to build and has been considered more difficult than tokamaks. This extra level of complexity also made it very hard to model on a computer, making it harder to predict and more expensive to build.
Spheromaks are similar to tokamaks but are somewhat different in the way they induce the magnetic field.
Compact toroids try to create fusion without a magnetic coil in the center of the torus (donut shape), reducing the need for complex magnets.
Lasers
Instead of squeezing hydrogen atoms together with a magnet, another approach using lasers tries to make them so hot that they collide with each other, which then instantly creates shockwaves pushing the hydrogen atoms together.
A good example is the U.S. National Ignition Facility (NIF), which guides, amplifies, reflects, and focuses 192 powerful laser beams into a target about the size of a pencil eraser. This delivers 500 trillion watts of peak power in one spot.

Source: Britannica
This is the other main design considered as likely to one day deliver viable commercial fusion.
Magnet-based fusion struggles with complex mathematics and superconductor material science. Laser-induced fusion struggles with delivering the energy properly and keeping the fuel dense and homogeneous enough for fusion to occur.
Electric Push
One last possible method to artificially achieve fusion is to use electric currents to generate the magnetic field squeezing the plasma tighter, or Magnetized Target Fusion (MTF).
One such method is the Z-pinch, another method uses pneumatic pistons and injection of plasma. A particle accelerator could maybe also achieve the same principle.

Source: IEEE
In general, these designs tend to be a lot more compact than tokamak or laser-based fusion.
Notably, it is the approach favored by the private fusion companies like General Fusion and Helion.
Steps To Commercial Fusion
Yields
As explained above, fusion is still very much an experimental field, with no obvious path toward a commercially viable design yet.
Overall, the yield of fusion reactors has been improving, meaning that they progressively start to yield more fusion energy from the energy injected into them to trigger the fusion.
In 2022, researchers at the US National Ignition Facility announced that they “created a reaction that made more energy than they put in”.
In practice, this claim is a little misleading: the laser-powered design indeed delivered 2.05 megajoules of energy and created 3 megajoules of energy of energy through fusion.
This ignores the fact that to create the 2.05 megajoules of laser, a total amount of electricity equal to 322 megajoules of energy was consumed to create these laser beams. So in practice, the total energy return is still 100x too small to make it a “real” positive return with that model. And even lower than that in practice because, for sure, not all of the heat generated could be converted back into power.
This is nevertheless an important milestone and an impressive achievement.
Plasma Stability And Reaction Duration
The key part will be to assess the situation of a self-sustaining fusion reaction, where the previous energy release is enough to trigger more fusion. Until recently, fusion reactions have lasted at best a few dozen seconds. In a future commercially viable reactor, such reactions could last dozens of minutes or even hours, thanks to more stable plasma.
This might be closer than many expected, with a new record of an entire 6-minute long fusion achieved by the WEST (tungsten (W) Environment in Steady-state Tokamak) device in France.
This illustrates how the innovative use of advanced materials like tungsten might pave the way for a drastic improvement over the classical fusion reactor designs. You can read more about tungsten and the hard-to-find investment opportunities in this sector in our article “Tungsten – The Secret High-Tech Metal”.
Cheap Superconductors
This step is needed especially for magnet-based fusion reactor designs but also for others, as the power levels usually require some superconductor materials to be used somewhere in the system.
Luckily, better superconductors, or even room-temperature superconductor technology, are progressing rapidly. You can read the details of that topic in our article “Progress In Superconductivity Making Way For A New Technological Revolution”.
AI
Plasma is an incredibly complex state of matter, very different from the other 3 (solid, liquid, gas). It is extremely hot and, overall, becomes quickly very unstable.
Unstable plasma tends not to stay confined for long in the reactor, interrupting the nuclear fusion process.
To compensate, nuclear reactor magnets try to constantly stabilize the plasma, adjusting the magnetic field in real-time. The mathematics associated with them are mind-bogglingly complex, and even supercomputers can struggle with them, especially if they need to perform them quickly to instruct the right reaction to the reactor’s magnet.
This could change, thanks to progress in AI, as we reported in a recent article. There, we explained how AI learned to predict the emergence of instabilities in the plasma up to 300 ms in advance.
“We no longer have to wait for the instabilities to occur and then take quick corrective action before the plasma becomes disrupted.”
Safety
Nuclear fusion is inherently much safer than nuclear fission. Fusion reaction automatically stops when the plasma expands, meaning there is no risk whatsoever of a runaway chain reaction.
However, before becoming a large-scale power source, nuclear fusion will still need to deal with a few safety issues:
- Many reactor designs use tritium, as these fusion reactions are easier to trigger than deuterium-deuterium fusion. However, tritium is radioactive, so any reactor failure could lead to (small) radioactive contamination.
- Plasma instability and high-energy physics carry an inherent risk. Keeping the operators safe and the reactor undamaged during continuous power-producing operations will require good safety procedures and probably design optimization.
- Nuclear fusion does occasionally produce neutrons, which will slowly turn the reactor wall into radioactive waste. While minimal in volume, these wastes will need to be properly processed at the end life of the components or of the reactors as a whole.
Afferent Topics
Space Fusion Propulsion
Currently, nuclear fusion is primarily pursued for its potential in power generation on Earth. Another sector that would greatly benefit from mastering nuclear fusion is space exploration and colonization.
Thanks to its very high efficiency compared to fuel mass, as well as extremely high temperatures, nuclear fusion reactors make for the perfect deep space propulsion systems.
In theory, it could deliver very quick acceleration and travel time, with low fuel requirement, and enhanced safety for the crew compared to alternatives like chemical or nuclear fission engines. The ease of access and overabundance of hydrogen in space are an extra bonus.
In practice, making a fusion reactor small and light enough to fit on a spaceship might be challenging, even after we master the design on Earth.
Would nuclear fusion become commercially viable, this would completely revolutionize the prospect of a space-based economy (which we discuss with and without fusion in our article here), and instantly make humankind a space-faring species.
Cold Fusion
Cold fusion is a controversial topic. In concept, this is the idea that nuclear fusion could be achieved without plasma at low temperatures.
A proposed method would be to use materials changing shape in such a way that hydrogen atoms are trapped and forced to fuse together. Hydrogen-infused metals like palladium, erbium, and titanium have been proposed to achieve it.
In 1989, researchers Stanley Pons and Martin Fleischmann claimed to have achieved such fusion. Unfortunately, years of trying to replicate the findings by the scientific community have so far been unsuccessful, leading to accusations of poor quality science or even outright fraud.
The following controversy would permanently damage the image of this concept. It is nevertheless still being worked on by a small number of scientists, usually under the names Low Energy Nuclear Reactions (LENR), Condensed Matter Nuclear Science (CMNS), or Chemically Assisted Nuclear Reactions (CANR).
A renewed interest in the field has occurred in the 2020s, looking to move past the stigma of unserious research. Notably, the US government agency ARPA-E announced in 2023 a handful of grants to fund research groups looking into low-energy nuclear reactions (LENR), following intriguing results achieved by NASA researchers in 2020.
Cold fusion is currently very uncertain and speculative. However, the return of serious and well-funded research to the field might clarify the situation and determine whether this could become a viable path toward achieving nuclear fusion.
Bubble Fusion
Another idea is that nuclear fusion could occur in bubbles when they collapse; for example, bubbles may form in water when submitted to ultrasounds, an idea also sometimes called sonofusion.
In theory, the shockwaves created by the collapse of a bubble in a liquid could be powerful enough to cause fusion, not completely unlike the way shockwaves induced by laser do. It could explain the phenomenon of sonoluminescence (the still not-understood emission of light when a bubble collapses).
The idea is as controversial as cold fusion, with its main promoter widely criticized.
However, the idea might not be as dead as the last two decades of controversy would make it appear.
In May 2024, a scientific paper titled “Observation of neutron emission during acoustic cavitation of deuterated titanium powder“, published in the ultra-prestigious journal Nature, claimed to have detected potential fusion events with bubbles of heavy water mixed with titanium particles.
We were able to sustain the neutron production for several hours and repeated the experiment multiple times under various conditions. We hypothesize that the observed neutrons originate from nuclear fusion of deuterium ions dissolved in titanium lattice due to the mechanical action of the impinging cavitation jets
The mix of a titanium lattice (like in cold fusion) with cavitation (bubbles) is more than intriguing, and the publication in a very serious peer-reviewed journal might reignite interest in the sector, with maybe “cold-bubble fusion” an unexpected scientific breakthrough.
Private Sector Entering In
Since its inception, the fields of plasma physics and nuclear fusion have mostly been driven by government research from public funding.
This makes sense, as they were highly useful for nuclear weapons development programs, with, for example, the U.S. National Ignition Facility initially developed to replace nuclear weapon tests more than explore nuclear fusion.
As a segment of science without direct commercial applications, funding for fusion had to be mostly from the public and academic sectors.
This is changing thanks to the convergence of 3 factors:
- Decades of experience in the sector have created a large body of free-to-access knowledge and trained scientists who can work for commercial companies.
- Nuclear fusion seems closer to being achieved commercially than ever before, increasing the enthusiasm of investors. And “moonshot” style of investments is now popular, with nuclear fusion maybe the ultimate moonshot together with asteroid mining, respectively permanently solving the problems of scarcity in energy and in raw materials.
- Climate change, geopolitics, and resource depletion are all converging to increase the demand for an abundant carbon-neutral source of energy.
So a new wave of nuclear fusion efforts is now driven by private companies, looking to rework reactor designs from first principles, investigate new methods, and trying to replicate for the fusion sector what companies like SpaceX have achieved for space flight (like the previously thought impossible rocket reusability).
Fusion Companies
Currently, none of the companies dedicated to making nuclear fusion commercially viable are publicly listed. This includes Helion, General Fusion, Commonwealth Fusion, TEA Technologies, ZAP Energy, and NEO Fusion. You can find an extensive list of startups in the nuclear fusion space on the dedicated page of Dealroom.
1. General Fusion
General is one of the startups leading the charge in making fusion a private sector venture, instead of a publicly-funded physics project.
The company was started as long ago as 2002, to develop Magnetized Target Fusion (MTF) technology.
MTF is expected by the company to be a shorter path to energy-positive fusion and to be a lot less costly. General Fusion was the first in the world to build and commission a compact toroid plasma injector at a power plant scale in 2010 and has reached many more milestones since.
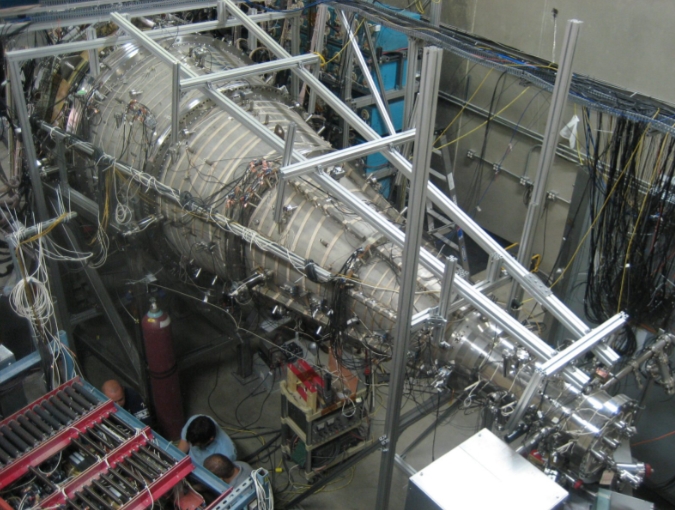
Source: General Fusion
The company aims to reach fusion with 100 million degrees Celsius temperature in 2025 and to progress toward energy breakeven (positive return from nuclear fusion) in 2026. Before that, a 1/5th scale model was made in 2023 and its performance matched the expectations from computer models.
Overall, General Fusion has spent 2 decades building step by step each of the core technologies of its final design, testing each along the way and successfully validating the idea, at least so far.
As a private company, it did not have to discuss and negotiate any design change, contrary to international projects like ITER. It could also pick technology on its own merit, without having to decide if a specific country should get the contract regardless of political reason.

Source: General Fusion
This is why many expect General Fusion and a few of its competitors to manage what large government projects might not.
2. Lockheed Martin Corporation
One notable exception to privately-listed startups dominating the field is the publicly traded company Lockheed Martin Corporation, a giant of the defense industry.
Lockheed used to work since the early 2010s on Compact Fusion, a nuclear fusion reactor it expected to be ready by the 2020s. However, it has since been announced that the work on the project was stopped in 2021.
The company has been very discreet about this project after an initial very public announcement. To this day, it is unclear what could have prompted the company to abandon the idea.
At the same time, it seems that it did not fully abandon the concept, notably with investments in 2024 in Helicity, a startup developing a fusion engine.
The idea would be to propel spacecraft with short bursts of fusion. Helicity is planning to use a plasma gun, the same approach as taken by General Fusion.
Potentially, Lockheed’s own internal results have shown that their design could not sustain fusion in a way that is compatible with energy production.
But maybe at the same time, short bursts are enough for the need for propulsion in space and much closer to becoming an actual product? It would also be a better fit with the overall aerospace and defense-focused profile of the company.
3. TAE Technologies
Formerly known as Tri Alpha Energy, the California-based company is focused on developing fusion energy tech. TAE Technologies is currently upgrading its fusion platform, Norman, to a sixth-generation machine called Copernicus.
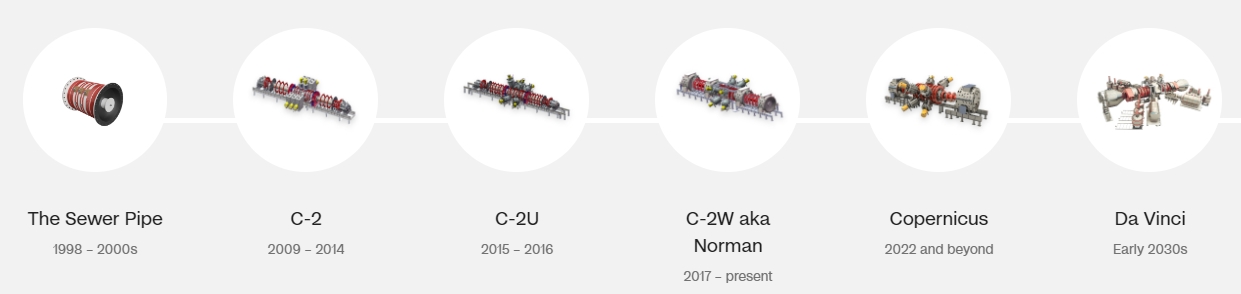
Source: TAE
TAE technology relies on particle accelerators to inject energy into the plasma and “act as a thickening agent that makes it more manageable”.
The company also extensively uses 3D printing in the making of Copernicus, allowing for quick iterations of new parts and quicker problem-solving. For example, it managed to print some reactor components for half the weight of what conventional manufacturing would have achieved.

Source: TAE
If all goes smoothly, the company expects to build its first prototype power plant that could connect to the grid in the early 2030s, scaling which to develop “robust and reliable” commercial power would continue through the decade. Fusion, according to its CEO Michl Binderbauer, would take us into a “paradigm of abundance.”
For the past 25 years, the company has operated on a “money by milestone” model, where each round of funding is only earned based on delivering on milestones that were promised to investors.
In 2022, Google and Chevron invested in TAE Technologies as part of the company’s $250 million funding raise. Google has actually been partnered with TAE for a decade now and provides the company with AI and computational power.
The company also offers life science services (Boron Neutron Capture Therapy -BNCT) and power solutions like batteries and e-mobility.
4. Helion
Helion aims to create fusion with deuterium and helium-3, instead of the more common approach to focus on fusion with tritium.
Normally helium-3 is very hard to find. But Helion has a method to produce it from deuterium in its own reactor. Otherwise, alternatives like unproven mining for it on the Moon would probably have been needed.
Like most private ventures in fusion, Helion uses plasma injection technology.
Another unique feature is targeting direct electricity capture from the plasma, using Faraday’s Law to induce a current, directly skipping the steam heating cycle common in nuclear power plants.
This move is rather bold, but also could increase the yield of future power plants by 2-3 fold, as heat-to-steam-to-power conversion is usually with a very low efficiency. It is also a very capex-intensive procedure.
Helion’s fusion power plant is projected to have negligible fuel cost, low operating cost, high up-time, and competitive capital cost. Our machines require a much lower cost on capital equipment because we can do fusion so efficiently and don’t require large steam turbines, cooling towers, or other expensive requirements of traditional fusion approaches.
Helion currently operates Trenta, its 6th generation reactor that has achieved 10,000+ pulses and 100 million degrees Celsius temperatures.

Source: Helion
It is currently moving to Polaris, its next model expected to push up to 100x quicker than Trenta, which would make it the first nuclear fusion to produce a net gain of electricity.
It is worth noticing that Polaris would be 19m long, far from a giant installation compared to other, more classic fusion reactor designs.